Monte Carlo simulations are extensively used to design radiation detectors and predict medical imaging system performance. Accurate modeling of radiation detectors, typically composed of a semiconductor or a scintillation crystal coupled to a photodetector, is required to predict performance. One of the basic principles of these detectors is the generation of a large number of optical photons (7,0000-60,000) when the incident high-energy particle interacts with the scintillator. The number of optical photons produced is proportional to the energy of the particle; efficiently collecting these photons is essential to achieve high performance of the detector.
Until recently, simulation tools used to study radiation detectors for nuclear medicine were designed for high-energy physics and only included simplistic models to track visible photons emitted in the scintillator. Since the spatial and temporal distribution of light defines the spatial, energy, and timing resolution of detectors, this is a huge limitation. We developed a new optical model, the “LUT Davis model”, based on measured 3D crystal surfaces and ray tracing with data stored in optical look-up-tables (LUTs). This work was validated in a series of benchtop experiments with positron emission tomography detectors and implemented in one of the widespread physics simulation software GATE (2000 users world-wide) in 2017.
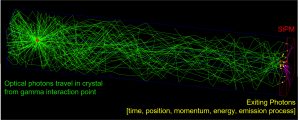
Example of an optical simulation in a scintillation crystal (blue) attached to a silicon photomultiplier (red).
We have applied this model to a variety of applications, including the study of depth-of-interaction detectors, and the time-of-flight detectors for positron emission tomography. We are now applying these simulation tools to advance the comprehension of Cerenkov light to design ultra-fast radiation detectors.
Publications
- Roncali et al, “Cerenkov light transport in scintillation crystals explained: realistic simulation with GATE,” Biomed.Opt. Express, 2019
- I. Kwon et al., “Dual-ended readout of bismuth germanate to improve timing resolution in time-of-flight PET,” Phys Med Biol, 2019
- Stockhoffet al., “Advanced optical simulation of scintillation detectors in GATE V8.0: first implementation of a reflectance model based on measured data,” Phys. Med. Biol., 2017
- Berg et al., “Optimizing light transport in scintillation crystals for time-of-flight PET: an experimental and optical Monte Carlo simulation study,” Biomed. Opt. Express, 2015
- Roncali et al., “Predicting the timing properties of phosphor-coated scintillators using Monte Carlo light transport simulation,” Phys. Med. Biol., 2014
- Roncaliet al.,“Simulation of light transport in scintillators based on 3D characterization of crystal surfaces,” Phys. Med. Biol.,2013.
Funding
NIH R03 EB025533 2015-2017
NIH R01EB027130 2019-2022
Contact
Emilie Roncali, eroncali@ucdavis.edu